SuCCESs Integrated Assessment Model
Overview
SuCCESs is a global Integrated Assessment Model (IAM) that focuses on representing the interactions between the energy, materials, land-use and climate systems. The model can be used to calculate global scenarios of these systems from 2020 to 2100, typically with the purpose of finding suitable strategies for mitigating climate change.
The model can be divided into four separate modules – energy, materials, land-use and climate – as portrayed in the figure below. Each module works under a distinct logic, as they represent very different kinds of systems. The interfaces between the systems establish the modules’ interaction with each other, e.g. how the greenhouse gas (GHG) emissions and sinks affect atmospheric GHG concentrations and thus the climate; or how land-use provides the raw materials and bioenergy for the energy and material systems. Each module is described in more detail in the sections below.
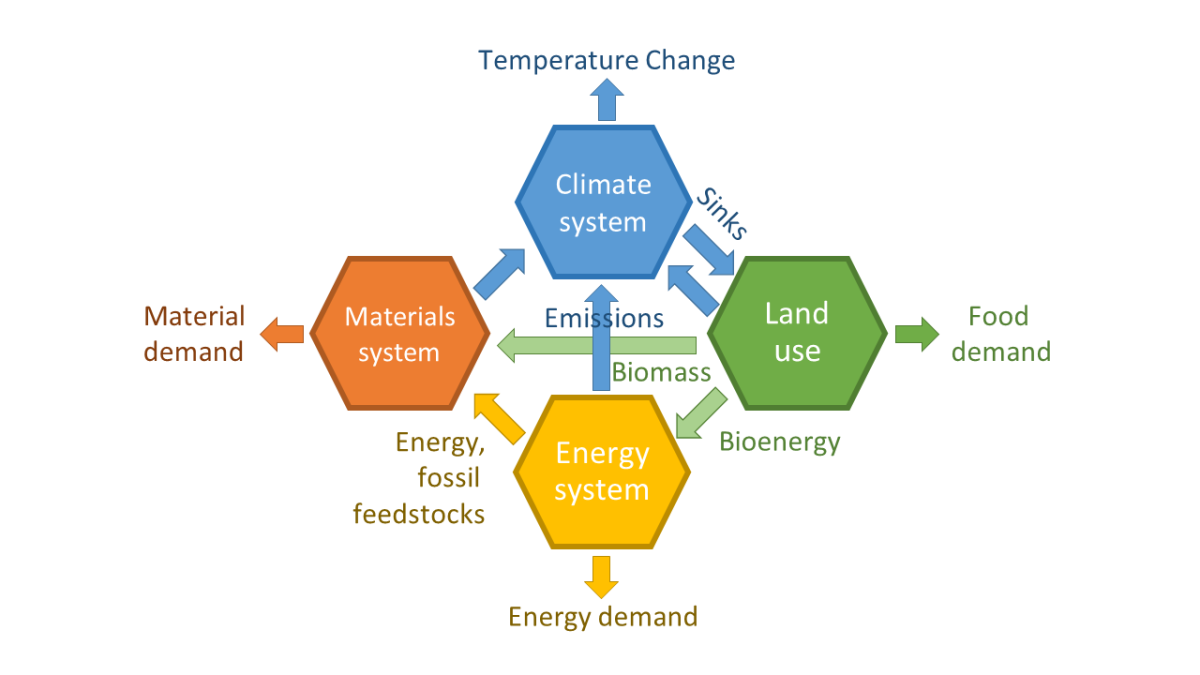
Technically, the model is a demand-driven partial equilibrium model, solved through intertemporal optimization (linear programming) with the objective to minimize the discounted costs from satisfying the projected demand for energy, food and materials, and also possible climatic and other constraints. Geographically, the model represents the world as a single region, although the land-use module CLASH separates between ten different regions (biomes) based on their characteristics for vegetation growth and terrestrial carbon stock dynamics. Temporally, the model represents variables on an annual level and runs in ten-year timesteps, although electricity production and consumption are represented also on an hourly level. The model is implemented in GAMS and is based partially on the OSeMOSYS modeling framework.
The main decision variables of the model represent the investment and operating decisions of production technologies, and the land area that is allocated to different land uses. Variables that follow from these decisions include the production quantities of different energy, material and food commodities, capacities of production technologies, GHG emissions and sinks from production and land-use, and aggregate measures of climate change (atmospheric concentrations of CO₂, CH₄ and N₂O, radiative forcing and the change in global mean temperature).
Energy and material modules
The energy and material modules portray the use and production of various energy and material commodities, and the investment and production capacity of technologies that process and produce these commodities. In this sense, there are two basic elements in the modules: commodities and processes. Examples of commodities in the model are crude oil, electricity, gasoline, steel and food crops. Examples of the processes in the model are wind turbine, oil refinery, blast furnace and passenger car.
Each commodity has a balance (an energy or a material balance), which requires that the production of the commodity needs to be greater or equal than the consumption of the commodity. (The balance can be also required to be equal for selected commodities.) Flows of commodities are mostly measures in energy (in PJ) or mass (in Mt), but for e.g. transportation the end-use commodities are passenger kilometers and cargo tonne-kilometers.
Processes describe specific technologies that produce the commodities, or more typically convert certain commodities into different commodities. A process is described through a set of parameters, including the ratios of input and output commodities (i.e. how much different inputs are needed to produce one unit of an output), capital and operating costs for investing into and running the process, emissions factors, capacity lifetimes, etc.
Together, the commodities and processes create a complex network. It starts from the extraction of primary commodities (e.g. crude oil, natural gas, wood, crops). These are then used as input to various transformation processes that produce secondary commodities. As an example, an oil refinery takes in crude oil and produces an assortment of petroleum products, including gasoline and light oil (diesel) for the transportation sector and naphtha and other feedstocks for the petrochemical industry. Typically, there are multiple steps in transforming commodities into another, from primary commodities to end-use commodities.
A projection of future demand is specified for the end-use commodities. This demand projection can be thought as the primary driver for the model: the demand needs to be met, and the optimization problem tries to find the least-cost way for satisfying the demand, while also satisfying the other constraints specified in the model.
The energy and material modules are based on the OSeMOSYS modeling framework, which provides basic accounting equations for energy and material balances, investments and production capacity, emissions from production technologies, and the accounting and discounting of costs.
Land-use module CLASH
Land-use is represented with the CLASH model (Climate-responsive Land Allocation model with carbon Storage and Harvests). CLASH models the allocation of land to different uses (cropland, pastures, managed forests, primary ecosystems, urban land), the stocks of carbon in terrestrial ecosystems, animal husbandry, the production of food crops, animal products and wood material; and GHG emissions from land-use.
In CLASH, the global land area is divided into 10 biomes, which are presented in the figure below. The biomes have different parametrizations for plant growth, carbon stock dynamics and crop yields. The parametrizations are based on the LPJ-GUESS dynamic global vegetation model run in different climate scenarios; and through this, the carbon dynamics and yields in CLASH react to climate change.
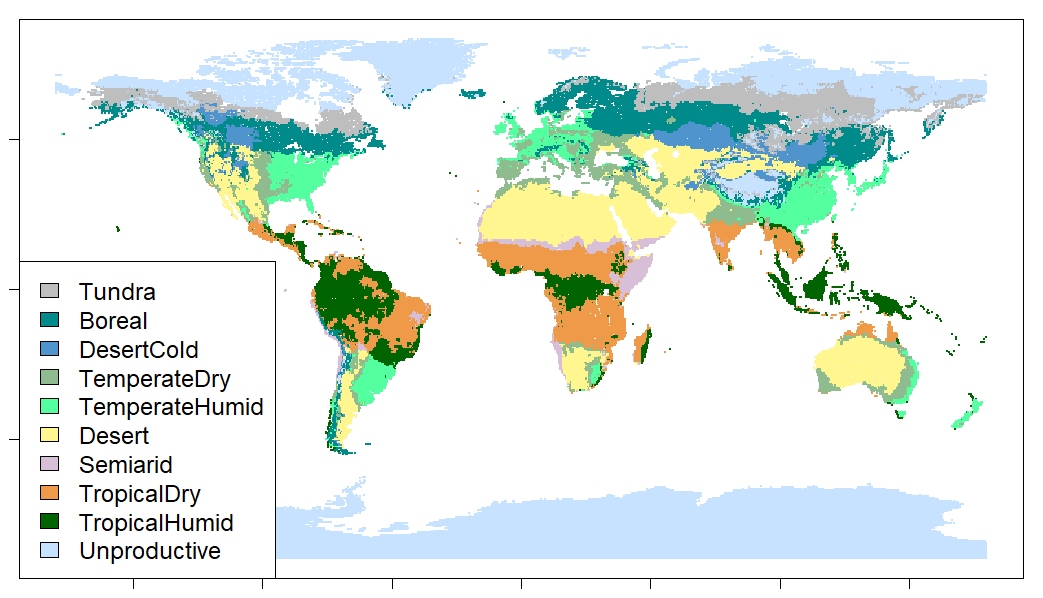
The main decision variable in CLASH is how much land-area in each biome is allocated to each land-use. Additionally, CLASH models managed forests by the age of the forest stands, and thereby it can decide at what age managed forests will be harvested. What results from these decisions is how much crops can be harvested from cropland, how much livestock products can be produced, how much wood can be harvested from forests, and how much carbon is stored in terrestrial ecosystems, including the vegetation, litter and soil carbon stocks.
The linkages of CLASH to the other modules arise from the production of crops and wood material, which can be used as energy in the energy module or as raw materials in the material module; and from the changes in terrestrial carbon stock and land-use emissions of CH₄ and N₂O, which affect the GHG concentrations in the climate module.
Climate module
The climate module provides a simplified representation of the climate system. It portrays how atmospheric GHG concentrations, radiative forcing and global mean temperature evolve, driven by the changes in GHG emissions and terrestrial carbon stocks in the other modules. The representation of the climate system is similar to those in many other IAMs – for example the DICE model – but differs in some of the details.
GHG concentrations are calculated for CO₂, CH₄, and N₂O. For CO₂, a four-box model is used to represent the exchange of CO₂ between the atmosphere and three ocean layers. This differs from e.g. the DICE carbon cycle representation in that the SuCCESs climate module omits the exchange between the atmosphere and the terrestrial biosphere, as the changes in terrestrial carbon stock are already modelled in the land-use module. A first-order decay representation is used for CH₄, and N₂O.
Increasing atmospheric GHG concentrations increase radiative forcing, the radiative imbalance in Earth’s atmosphere. We use linearized versions of the simplified expressions for radiative forcing from CO₂, CH₄, and N₂O concentrations presented in the IPCC AR5.
Radiative forcing leads to an increase of mean temperature in the atmosphere, which exchanges heat with the oceans. We use a two-box model to represent this. However, the propensity for the climate to warm following the increase in GHG concentrations is relatively uncertain. We use the ‘best-guess’ value of 3.0°C for climate sensitivity, which represents the long-term equilibrium in global mean temperature increase following a doubling of CO₂ concentration from the pre-industrial level.
The climate module of SuCCESs serves two roles. On one hand, it provides a simplified way to represent climate change within the optimization model. This enables the calculation of scenarios that have a constraint on the temperature increase, for example to the 1.5°C or 2°C levels described in the Paris Agreement. On the other hand, it can provide the climate scenario (CO₂ concentration and temperature increase) for CLASH, which is used to parametrize vegetation growth, yields and carbon stock dynamics.
In this role, however, it is important to note that parametrization needs to be run separately, as it is not changed endogenously within a single model run. The reason for having the CLASH parametrization as exogenous is that it would lead to a non-linear model formulation, with the climate variables being used in the parameters, which act as coefficients to other variables. As SuCCESs is formulated as a linear programming problem (to harness the major advantage in computational efficiency of linear programming algorithms compared to non-linear ones), such an endogenous parametrization would not be possible. However, if the model is run with a climatic constraint, for temperature change, radiative forcing or emission trajectories; CLASH can be pre-parametrized with reasonable accuracy. Alternatively, SuCCESs and CLASH parametrization can be run iteratively, until sufficient convergence between the parametrization and the climate scenario is attained.
Model outputs
The results from SuCCESs are long-term scenarios within the specified module domains. The result variables include e.g. the following:
Production, consumption and flows of energy and material commodities
Investments, capacities and operation of production processes
Land-use allocation to different uses
Production of agricultural and forestry products
GHG emissions from energy, material production and land-use
Changes in terrestrial carbon stocks (vegetation, litter and soil)
Climatic variables (GHG concentrations, radiative forcing and temperature increase)
Shadow prices of commodities, emissions and land-area
The figures below illustrate some example results from the SuCCESs IAM:
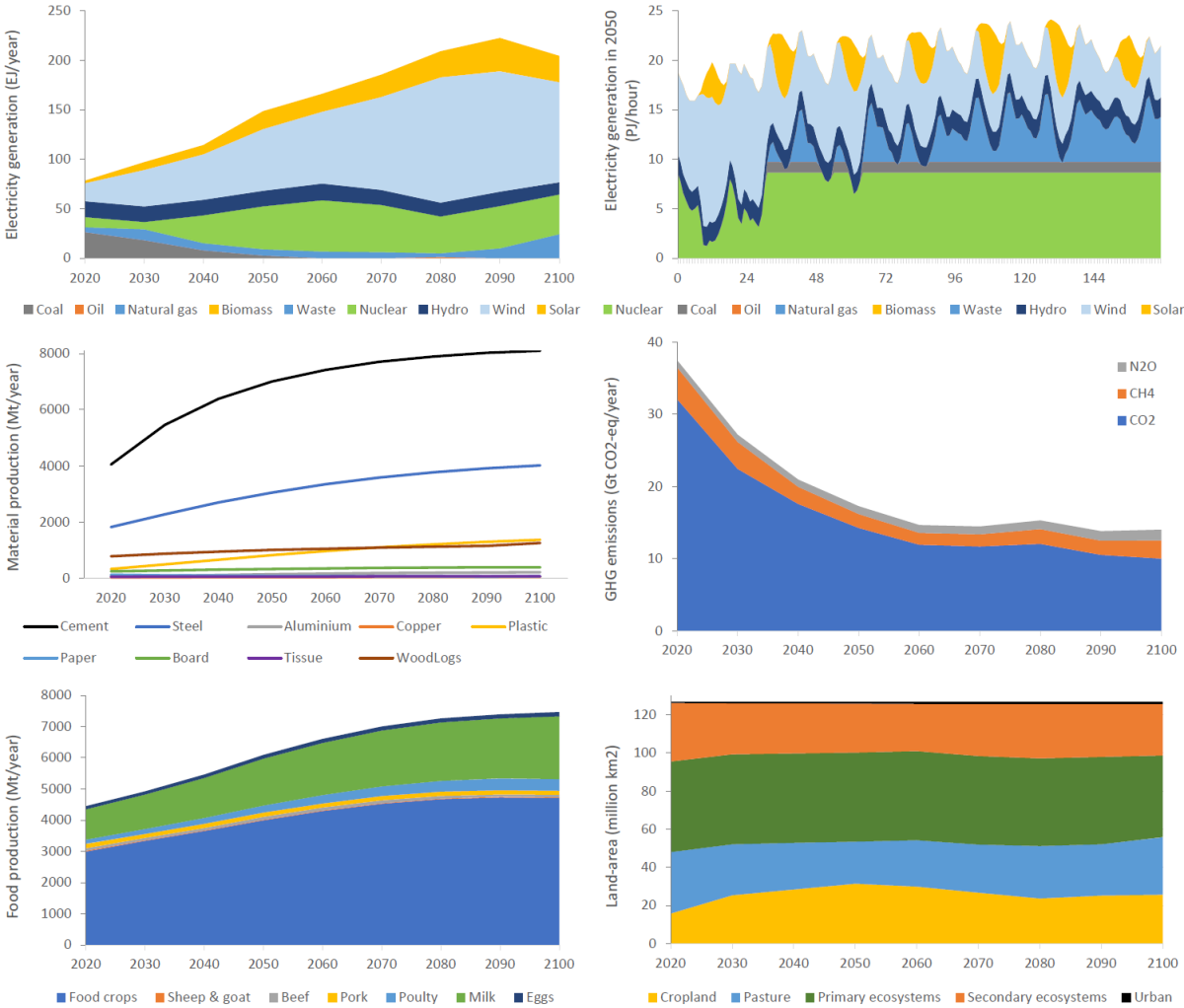